Lithium-ion battery separators – crucial patent developments
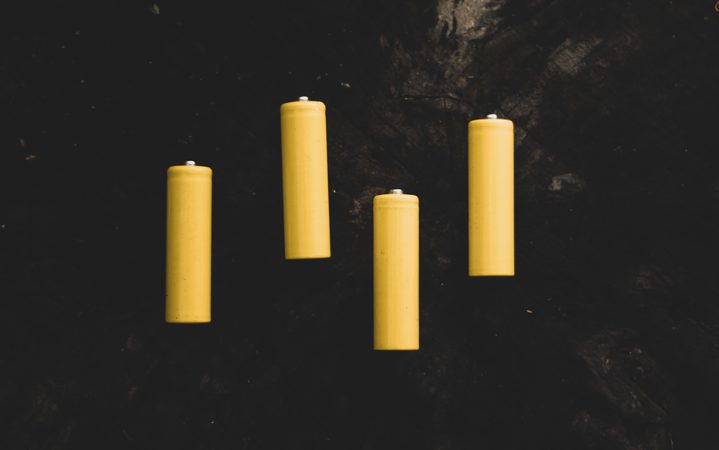
The properties and characteristics of a lithium-ion battery separator are vital for performance and safety. Here, we analyse recent patent activity and examines Morgan Advanced Materials’ important new patent, which relates to ionic conducting compositions for energy storage devices.
Lithium-ion — the fundamentals
The lithium-ion revolution was born in the early 1990s in response to the demand for smaller and more powerful batteries as compared with nickel–cadmium (NiCad) batteries. The battery used in Sony’s Camcorder needed to last longer and be made more compact — a challenge that was overcome, making the product widely successful [1].
Lithium-ion batteries have four main components: an anode, a cathode, an electrolyte and a membrane or separator. The role of the separator is to provide a barrier between the two electrodes and in a liquid electrolyte reservoir it needs to be electrically insulating but permeable to lithium ions.
Characteristics of battery separators
The development of separators exhibiting optimum properties is challenging. This is due to the complexity of the variables involved and their interdependencies. In addition to the performance criteria desired by industry (and in some cases, bespoke industrial applications) there is still a demand for separators that are lighter, more compact and cost-effective.
The suitability of a separator as a barrier is often correlated to its thickness and mechanical and dielectric strength — separators must be sufficiently thick to prevent short circuits otherwise caused by dendritic growth of the lithium metal. Ion conduction is heavily influenced by its air permeability, tortuosity, pore size and pore distribution. As a reservoir for an electrolyte, its porosity and wettability are also important.
Ultimately, the properties and characteristics of a separator are vital for achieving both battery performance and safety — for example, the chemical stability of a separator will affect the safety and cycle life of a battery, while its thickness and pore size will affect the battery capacity and power.
Industrial demand
The industrial demand for high-energy-density lithium-ion cells has led to a corresponding surge in interest for separators that provide high performances for various product types and cell sizes with improved safety and reliability. The growth appears to be due to rising demand in the electric vehicles sector but may also be attributed to energy storage systems used in the green energy sector, including renewable energy storage.
Global markets including electronic devices, power tools and electric vehicles have experienced rapid growth. Demand for separators in these fields continues to increase (in area), from ~900 million m2 in 2015 to an estimated ~3000 million m2 by 2025 [2]. Other emerging fields, including commercial electric aircrafts, may further add to this demand. Last year, Asahi Kasei Corporation responded to the booming global demand in the battery market with an investment of ~€238m to expand its production of lithium-ion battery separators (in area) from 450 million m2 to 1.5 billion m2 by 2021.
Competition in the lithium-ion battery separator market is fierce. Some of the largest suppliers are Asahi Kasei (24%) and Celgard (11%), Toray (21%), SK (11%), UBE (9%), Sumitomo (7%) and others (17%). The market trends are evident, and all the big players are protecting their innovations to capitalise on market share through patent filings as the industry prepares for the mass production of new battery technologies.
Patent filing activity
The number of patent filings relating to separators for use in lithium-ion batteries alone has significantly increased in recent years. As new industries and applications such as electric vehicles are being established, new technological advances like solid-state batteries are being made in response to the forecasted growth. As the reliability of these new technological developments improves and performance criteria are met, confidence in the market is being affirmed. Existing and new manufacturing facilities are being prepared and this will ultimately clear the way for commercialisation, which needs scalable technologies supported by facilities for mass production.
Solid-state electrolytes — the IP landscape
Liquid electrolytes, which are most usually flammable carbonates, pose the risk of a high frequency of catastrophic failure. An increasingly popular alternative to lithium-ion cells with liquid electrolytes are those incorporating solid-state electrolytes. These allow the use of lithium metal as an electrode with the only risk of dendritic growth being a ‘soft short’ — a cell failure without an associated fire. Solid-state electrolytes replace both the liquid electrolytes and the separators. Several categories of solid-state electrolyte materials have merit, the most common being oxides, sulphides and polymers, although challenges for oxides and sulphides include reactions with air and moisture. Cells including polymers need to be operated above room temperature and charged slowly.
The IP landscape for solid-state battery technologies appears to be a minefield. The number of patent publications per year relating to solid-state batteries has dramatically increased in recent years, with applicants including Toyota, Murata, Panasonic, Google, I-ten, and Sakti3.
Patent of the month — Morgan Advanced Materials
In this month’s issue of the Materials World magazine, I discuss a recently granted UK patent relating to ionic conducting compositions for energy storage devices.
The patent (GB2568613B) was granted in June 2020 to Morgan Advanced Materials PLC— a global manufacturer of specialist products, using carbon, advanced ceramics and composites for a broad range of markets.
The patent discloses an energy storage device comprising a melt-formed glass or glass ceramic silicate composition comprising the formula LivM1wAlxSiyOz where M1 is selected from the group consisting of alkaline earth metals, Ti, Zr, Ce, La and combinations thereof; v, y, and z are greater than 0; w is greater than 0; x is 0 or greater than 0; y ≥ x; and wherein the formula <u
- accounts for at least 95 wt.% of the composition and wherein the lithium content (expressed as </u
- Li2O) is less than 15 wt.% of the composition. Optionally, M1 may include Zr, which was found to enhance the ionic conductivity of the composition.
The novel material exhibits a high enough conductivity for use as a stand-alone electrolyte or within a polymer matrix in solid-state electrolyte cells. The silicate composition is melt formed — i.e. it is formed from a molten mass using melt techniques including those suitable for forming fibres such as bushing, blasting, blowing and spinning techniques. These processes, in which the silicate composition is formed as fibres or nanowires, allows for the manufacture of these materials on a larger scale as compared with that of conventional materials made using sol-gel or similar techniques. The material is also stable for processing in air and can be scaled-up to mass production at an acceptable price to the lithium-ion battery industry.
There are various possible failure mechanisms for lithium-ion batteries and this patent is only one of the recent developments by Morgan Advanced Materials in relation to improving the safety, reliability and performance of energy storage devices.Failure in a lithium-ion cell containing a liquid electrolyte can result in a rapid rise in temperature known as ‘thermal runaway’, which would cause the electrolyte to ignite. Thermal runaway of one cell causes a chain reaction through adjacent cells, leading to an explosion of the battery pack. Morgan Advanced Materials is taking industry-leading products and expertise in developing thermal management systems (which have transformed gas and diesel-powered trains to electrified trains) and is now applying that capability to the automotive sector.
For advice on protecting innovations in materials science and engineering, our dedicated chemistry and materials technologies team is here to help.
[1] Clark, R., Morgan Advanced Materials plc (2019), SOLBAT – Challenges and Opportunities from an Industrial (Materials) Perspective, Faraday Institution Meeting, 21 February 2019
[2] Clark, R., Morgan Advanced Materials plc (2016), Improving Safety in Lithium-ion Batteries using Thermally Stable Separators including Ceramic Coatings, Ceramics Expo, 26 April 2016
[3] Morgan Advanced Materials plc 2020, Managing heat more efficiently and effectively is the new ‘must have’ of next generation hybrid and electric vehicles, viewed 28 September 2020